Acid-Base Transesterification of Oil with High Free Fatty Acid Content Kumar Anil1,*, Valoyi Redeem2, Ochieng Aoyi3, Onyango Maurice4 1Department of Chemical and Process Engineering, Moi University, Kenya 2School of Chemical and Metallurgical Engineering, University of the Witwatersrand, South Africa 3Department of Chemical Engineering, Vaal University of Technology, South Africa 4Department of Chemical and Metallurgical Engineering, Tshwane University Technology, South Africa *Email ID: akumar@mu.ac.ke
Abstract Biodiesel has emerged as one of the alternative biofuels to non-renewable fuels, and the typical method of producing biodiesel is by transesterification process. This process was employed in the present work to synthesize biodiesel from waste vegetable oil using acid and base catalysis methods to improve the yields and to identify the optimum operating conditions. Response surface methodology was used to determine the optimum operating parameters. The free fatty acids (FFAs) in the oil were reduced using acid catalyst (H2SO4 before a base catalyst (NaOH) was added. The duration for acid catalyzed process was determined such that it was just short enough to esterify the FFAs that were in the oil. The subsequent base catalyzed synthesis results showed that biodiesel was formed and the highest biodiesel yield was at 2% weight catalyst, 50°C and reaction time of 1.5 h. A further increase in temperature at 2% catalyst weight favoured the formation of soap and had a negative impact on the yield of biodiesel. The yield increased with an increase in temperature, catalyst weight and reaction time. The highest yield was at 3% weight catalyst, 60°C and reaction time of 2 h. The results of the acid-base catalyzed synthesis showed that biodiesel yield was improved as compared to the base catalyzed synthesis alone due to the fact that acid catalyst was responsible for esterifying the FFAs, and subsequently when the base catalyst was added, more biodiesel was formed because no soap formation took place in the reaction. The kinematic viscosity of acid-base catalyzed biodiesel satisfied the standard test method for kinematic viscosity (ASTM D445). Top Keywords Biodiesel, Waste vegetable oils, Transesterification, Acid-base catalysis. Top |
Introduction Biofuels have recently emerged as alternative fuel to non renewable fuels. The current major biofuels are fuel ethanol and biodiesel. Two methods of biodiesel production are enzymatic [1, 2] and transesterification [3, 4] processes. The main limitation of the enzymatic process is the cost of enzyme (lipase), which can make the process uneconomical. In transeterification process, biodiesel is produced from a reaction between vegetable oil and an alcohol in the presence of a base or acid catalyst. The alcohols that can be used in the transesterification process are methanol, ethanol, propanol, butanol and amyl alcohol [4, 5]. Methanol and ethanol are the most frequently used alcohols, but methanol is often preferred over ethanol because of its low cost and its physical and chemical advantages whereas ethanol is not frequently used because it forms an azeotrope with water which makes it difficult for separation process. |
The first generation biodiesel has been produced from edible vegetable oils such as sunflower oil [6] cotton seed oil [5]. However, the use of edible feed stock causes a threat to food security, and for this reason, more recently the second generation biodiesel has been produced from non-edible raw materials such as waste vegetable oil [7,8] and Jatropha curcas oil [1,9]. Most non-edible oils and some types of lipids have high content of free fatty acids such as phospholipids, sterols, water, odorants and other impurities, and these influence the quality of the product [10]. In the homogeneous acid and base catalyzed transesterification processes, the most commonly used base catalysts are NaOH and KOH, while acid catalysts include sulphuric acid and sulphonic acid. The main advantage of the base catalyzed transesterification over the acid one is that it is fast and can be conducted at low temperatures (303–338 K) and pressure (0.1 MPa). The acid catalyzed method has the advantage that it leads to high biodiesel yield. However, the reaction is so slow that one run can take more than one day. The two major challenges are: the use of acid catalyst poses a problem of slow reaction whilst with base catalyst saponification reduces the yield. In this work, waste vegetable oil was used to synthesize biodiesel using simultaneous acid-base catalysis method to improve the yields and to identify the optimum operating conditions. |
Top Materials and Methods Reactions were carried out in a 200 ml batch reactor equipped with a reflux condenser and a mechanical stirrer. The reaction temperature was controlled using a constant-temperature water bath. The oil used for the production of biodiesel was sampled and analyzed. The free fatty acid (FFA) contained in the oil was estimated by titration method. The catalyst was weighed and then mixed with methanol in a conical flask while stirring to allow sodium hydroxide to completely dissolve in methanol to form sodium methoxide. In order to initiate the transesterification reaction, oil was pre-heated to the desired reaction temperature on a hot plate and thereafter the sodium methodoxide was added to the pre-heated oil. The reaction was allowed to continue at the desired temperature for a specific period of time. After the reaction time elapsed, the reaction mixtures was transferred into a separating funnel and allowed to cool and separate into two product layers. The products of the transesterification reaction are biodiesel, being the top layer and the bottom layer contained glycerol, residual methanol, trace water, and salts. The bottom layer was drained, weighed and thereafter heated to 70°C on a hot plate in the fume hood in order to remove the residual methanol and water. The top layer of biodiesel was weighed then washed with water to remove traces of salts and methanol. After washing the biodiesel, it was air dried to remove moisture and then reweighed. The infra-red spectrometer was used to measure the content of biodiesel and glycerol. A 33 factorial (three factors, each at three levels) experimental design and response surface methods were used to optimize the biodiesel synthesis process. The responses selected were biodiesel product yield, density and kinematic viscosity, while the factors were reaction temperature, time and catalyst amount. |
Operating Conditions The operating conditions investigated were temperature, reaction time, amount of catalyst. The product yield and kinematic viscosity (responses) were calculated using Reynolds Stress Model (RSM) equations modelled on the experimental results. The three input factors that were investigated in this experiment were catalyst weight, temperature and reaction time of the acid stage process. A two level factorial method with all input factors set at two levels was used (Table 1). These levels are referred to as ‘high’ and ‘low’ and they are denoted by ‘+1’ and ‘-1’, respectively. The two level factorial design method is designated by 2k, where the number refers to the level and the letter k refers to the number of input factors. The 2k factorial method assumes linearity in the factor effects and when the linearity assumption in the factor effects fails, significant experimental errors will be possible. Hence, there is method of replicating certain points in a 2k factorial method that will provide protection against non linearity and the method involves adding a centre point to the 2k factorial design. The 2k factorial design with a centre point requires a centre point to be added and replicated at least four times which makes the number of runs for the experiment to be 12 (23+4=12). Table 1 shows level variation of input factors using 23 factorial design method with a centre point and these operating conditions were used for capturing data during the experiment. Waste Vegetable Oil Titration methods were used to determine the percentage of FFA present in the oil. The waste vegetable oil (WVO) was first mixed with isopropyl alcohol and then a mixture of 0.1M NaOH in water was added until all the FFA was neutralized. The point that confirms that all the FFA had been neutralized was indicated by the pH of the mixture during titration. A pH of about 8.5–9 signified that all of the FFA has been neutralized. The dynamic viscosity of biodiesel was measured using Ostwald viscometer. Top Results and Discussion The amount of FFA determined by the titration method was used to estimate the molecular weight of WVO [11], which was found to be 873.41 g/mol. This is in agreement with the average value of 866 g/mol reported by Cetinkaya and Karaosmanoglu [11]. The product was then analyzed using IR spectroscopy which revealed the presence of the key hydrocarbon groups. |
Transesterification Figure 1 shows the infrared analysis of reaction mixture for acid catalyzed biodiesel and there are four strong peaks displayed in the figure. The first peak has medium peak intensity with wavelength values of 729.14 cm−1 and the second peak has wavelength values 1168.84 cm−1. The first peak represents the C-H alkene bond group while the second peak represented the bond type of methyl esters, R-COOCH3. The third peak has a wave length of 1747.47 cm−1 which corresponds to the bond type of C=0 esters and the fourth peak has a wave length of 2925.96 cm−1 which correspond to the bond type of C-H alkanes group. The formation of ester products was confirmed by the Fourier Transform Infrared Spectroscopy (FTIR) analysis (Figure1) which showed the presence of ester product in the reaction mixture. Esterification The effects of acid catalyst weight were studied at the reaction conditions of 40°C, 50°C and 60°C. Figure 2 and 3 show that the esterification reaction was dependent on the reaction temperature with the highest esterification or lowest FFA obtained at 60°C after 210 minutes. Figure 2 shows that the FFA at 60°C was as low as 0.43% after 210 minutes and at the temperature of 50°C was found to be 0.50%, while at the temperature of 40°C the FFA was found to be at 0.64%. Figure 3 shows that the FFA at 60°C was as low as 0.54% after 210 minutes and at the temperature of 50°C the FFA was found to be 0.73%, while at 40°C it was found to be 0.92%. However the similarity between Figures 2 and 3 is that the results show that esterification reaction was dependent on temperature and increased with increasing temperature. Base catalyzed Synthesis Table 2 shows the standard experimental matrix for the factorial design and also shows the results of the biodiesel yield and viscosity. Equation (1) describes the influence of catalyst weight and temperature at a fixed methanol to WVO molar ratio on biodiesel yield within the studied experimental ranges. Where x1, x2 and x3 are the levels of catalyst weight, temperature and reaction time respectively, xij represents the interaction between factor i and j. Equation (1) can be represented as three dimensional response surface plots for predicted values of biodiesel yield over the studied experimental range of catalyst weight and temperature (Figure 4). A statistical analysis was carried out with experimental values and The RSM model equation (1) was checked for reliability by the unbiased estimator (ό2) and the coefficient of determination (R2) were found to be 6.22xl0−5 and 0.9512, respectively. Figure 5 shows the biodiesel yield RSM plot of base catalyzed synthesis at Temperature of 50°C with varying reaction time and catalyst weight. Increasing catalyst weight at a constant level of reaction time does not have much effect on biodiesel yield, but increasing catalyst weight at constant level of reaction time decreases biodiesel yield. The behaviour of catalyst weight and reaction time interaction shows that reaction time does not have much effect on biodiesel yield. The low value of the unbiased estimator (ό2) shows that the RSM model well predicts the experimental values attained in the laboratory and the value of the coefficient of determination is closer to 1 which indicates that the RSM model fits the data. Figure 4 shows the three-dimensional response surface plot of the biodiesel yield predicted for the experimental range of catalyst weight and temperature at fixed methanol to WVO molar ratio of 6:1. The yield decrease with both increase in temperature and catalyst Acid and Base Catalyzed The bio diesel yield for acid and base catalyzed synthesis was also analyzed using surface response method. Equation 2 describes the influence of catalyst weight and temperature at a fixed methanol to WVO molar ratio on biodiesel yield within the studied experimental ranges. Figure 6 shows the three-dimensional response surface plot of the biodiesel yield predicted by equation (2) for the experimental range of catalyst weight and temperature. Statistical analysis was carried out with experimental values and the RSM model equation (2) was checked for reliability by the unbiased estimator (ό2) and also the coefficient of determination (R2) was calculated. The increase in catalyst weight at low temperatures (Figure 6) resulted in an increase in the biodiesel yield, which is consistent with the work of Vicente et al. [3]. However, the increase of catalyst weight at higher temperatures above 50°C shows that the biodiesel yield starts to decrease. The decrease in biodiesel yield at higher catalyst weight and higher temperature can be attributed to the formation of soap which hinders the production of biodiesel. The formation of soap or saponification reaction is an undesirable side reaction which is favoured by higher temperatures. The unbiased estimator (s2) was found to have a value of 9.004X10−4 and the coefficient of determination (R2) was 0.9862. The low value of ό2 shows that the RSM model predicts the experimental values attained in the laboratory and the value of R2 is closer to 1 which indicates that the RSM model adequately fits the data. There was an increase in biodiesel yield with an increase in catalyst weight and temperature. This trend (data not presented) is contrary to that in Fig. 4, where the biodiesel yield decreases at higher temperature. The increase of biodiesel yield at higher temperature of acid-base catalyzed synthesis is due to that the acid catalyst was responsible for esterifying FFAs that are responsible for the formation of soap at higher temperatures. Top Conclusion Biodiesel was produced from waste vegetable using base and acid-base transesterification method. The results showed that the properties of biodiesel produced were comparable to the commercial one. The highest biodiesel yield was at 2% weight catalyst, 50°C and reaction time of 90 minutes. Further increase of temperature at 2% catalyst weight favoured the soap formation and had a negative impact on biodiesel yield. Under these studied conditions, acid catalyzed synthesis did not produce quantifiable amount of biodiesel, but the effect of the acid catalyst was more active in esterifying free fatty acids that were in the oil. However the acid-base catalyzed synthesis results showed that biodiesel yield was improved as compared to the base catalyzed synthesis due to the fact that acid catalyst was responsible for esterifying the FFAs and when the base catalyst was added more biodiesel was formed because no soap formation was taking place in the reaction. The biodiesel yield increased with increase of temperature, catalyst weight and reaction time. The highest biodiesel yield was at 3% weight of the catalyst, 60°C and reaction time of 120 minutes per catalyst. The kinematic viscosity of acid-base catalyzed biodiesel satisfied the ASTM D445 Standard |
Top Figures Figure 1.: IR spectroscopy of acid catalyzed transesterification
| 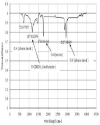 | |
| Figure 2.: Temperature variation of esterification at 0.5% weight catalyst
| 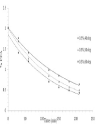 | |
| Figure 3.: Temperature variation of esterification at 1% weight catalyst
| 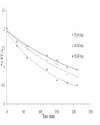 | |
| Figure 4.: RSM plot of base catalyzed synthesis at reaction time of 90 minutes
| 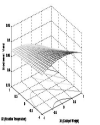 | |
| Figure 5.: Biodiesel yield RSM plot of base catalyzed synthesis at Temperature of 50°C
| 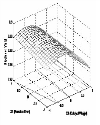 | |
| Figure 6.: RSM plot of acid-base catalyzed synthesis at reaction time of 90 minutes
| 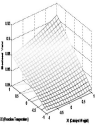 | |
|
Tables Table 1:: Operating parameters
| Level | Facto | Catalyst, g | Temperature,°C | Reaction time, min | -1 | 100.0 | 45 | 10 | 0 | 100.0 | 60 | 20 | +1 | 98.0 | 75 | 30 |
| | Table 2:: Factor levels for a 23 factorial design for acid-base catalyzed synthesis
| Run no. | Acid mass (g) | Temperature level (°C) | Reaction time level (min) | 1 | +1 | -1 | -1 | 2 | -1 | +1 | -1 | 3 | +1 | +1 | -1 | 4 | -1 | -1 | +1 | 5 | +1 | -1 | +1 | 6 | -1 | +1 | +1 | 7 | +1 | +1 | +1 | 8 | 0 | 0 | 0 | 9 | 0 | 0 | 0 | 10 | 0 | 0 | 0 | 11 | 0 | 0 | 0 |
| |
|