Introduction With rapidly increasing environmental concern, the stakes are exceedingly high for the search of a greener substitute for various petrochemical products including fuels. However, there is almost unanimous opinion among both proponents and skeptics that the potential of the greener substitute requires attention to both safeties as well as economical issues. In this context, global biodiesel industry has recently experienced a major surge worldwide. Some of the main drivers behind this tremendous growth are reducing dependence on imported oil, environmental friendly alternative to diesel and reducing greenhouse gas emission [1], The euphoria once associated with biodiesel production has given way to disappointment due to lack of economic viability as well as enormous amount of waste glycerol generated in its preparation. The current annual amount of glycerol arising from this biodiesel production amounts approximately 1.9 Mtons and will continue to rise proportionally. Germany, one of the principal biodiesel-producing countries has started treating glycerol as an “industrial waste” since 2007 [2]. The crude glycerol contains too many contaminants like unconverted free fatty acids, methanol, biodiesel and soaps making it unfit for any useful applications in chemistry and pharmacy without purification, moreover, the high purification cost of glycerol makes it application limited. Thus, the benefits and opportunities associated with biodiesel production can be efficiently reaped by capitalizing upon the value added products that this waste glycerol can generate. |
At current prices (2.5 cents/lb), glycerol is very competitive with sugars used in the production of chemicals via microbial fermentation [3]. The most obvious target of glycerol conversion and valorization by the means of fermentation technology is its biotransformation in to 1,3-propanediol. 1,3-propanediol has attracted world wide attention due to its enormous applications in polymers, cosmetics, foods, adhesives, lubricants, laminates, solvents, antifreeze and medicines [4,5]. More importantly, 1,3-propanediol is a critical copolymer ingredient in a new textile fiber that is being marketed for its “green” nature and also because it provides properties relative to other commercial textile fibers [6]. The natural producers of 1,3-propanediol from glycerol are of genera Klebsiella, Clostridia, Citrobacter, Enterobacter and Lactobacilli. Although utilization of crude glycerol in the fermentation medium without prior treatment offers a remarkable advantage against the traditional use of pure glycerol as a substrate, but still it is hard to escape from the general conclusion that the presence of certain impurities makes it unsuitable for microbial growth and fermentation. In fact, only few researchers have recently investigated the potentials of crude glycerol obtained from biodiesel processes for 1,3-propanediol production [7,8]. The majority of the studies for microbial production of 1,3-propanediol have been conducted using pure glycerol. In this study, we have evaluated the potentials of our selected strain of Citrobacter freundii for 1,3-propanediol production from glycerol (crude and commercial). To the best of our knowledge, there is no report on utilization of crude and pre-treated glycerol obtained from biodiesel process using C. freundii. One of the objective in this study was to evaluate the potential toxic effect on growth and 1,3-propanediol production by C. freundii by the utilization of crude and pretreated glycerol. The present work also provides a process for treatment of crude glycerol using hexane prior to fermentation to get rid of most of the impurities. Several physiological and nutritional factors were optimized using “one-variable-at-a-time” method (detail results not presented here). The process was scaled up to 30 L fermentor in which the final yield of 1,3-propanediol reached to maximum of 28.Og/1 under anaerobic condition and then the results compared with the existing 1,3-propanediol producing strains. |
Top Materials and Methods Biodiesel Synthesis Transesterification of different oils (Jatropha, JT; Soyabean, SB; Sunflower, SF; Kardi, KR; Linseed, LN; Rice bran, RB) were carried out with pure methanol in a molar ratio (oil:methanol) of 1:4 using 0.5% (w/v) NaOH as a catalyst. First, NaOH was added to methanol, stirred until the catalyst was completely dissolved, then mixed with 1 L of oil and incubated at 450C at 200 rpm for 12 hr under shake flask condition. These conditions were already optimized in our laboratory. Following completion of the reaction, the product was kept in a separatory funnel to allow the separation of two phases. Crude glycerol was obtained directly from the bottom phase without any purification and used for further investigation. Microorganism and Growth Conditions In the present investigation, 1,3-propanediol producing Citrobacter freundii was used. This organism was grown in 500ml anaerobic bottles containing 200ml of the medium which consisted of (g/L): Glycerol, 50; Glucose, 1.25; K2HPO4.3H2O, 5.0; KH2PO4, 3.48; (NH4)2SO4, 2.0; MgSO4.7H2O, 0.4; CaCl2.2H20, 0.2; Mg2CO3, 10; CoCl2.6H20, 4.0 mg, tryptone, 15.0, at pH 7.0. Glycerols used in the above medium were obtained from three different sources i.e pure commercial glycerol (99% w/w); crude glycerol obtained from biodiesel preparation; pretreated glycerol after solvent washing. The medium was sealed with butyl rubber bungs with N2 headspace. The N2 headspace was replaced with CO2. The medium was sterilized for 15 min, at 121 0C. To remove traces of dissolved, oxygen, Na2S.9H2O (0.02% v/v) was added. The reduced medium was then inoculated with 2.0% (v/v) seed inoculum (0.7–0.8 O.D. at 660nm). Inoculum was raised at 37 ± 10C in 250 ml shake flasks containing 50 ml of preculture medium at 150 rpm under aerobic conditions. Preculture medium contained (g/1): Beef extract, 1.0; Yeast extract, 2.0; Peptone, 5.0; NaCl, 5.0 at pH-7.0. Solvent Washing Crude glycerol obtained from biodiesel preparation was washed with hexane (1:1 ratio) at 200 rpm for 3 hr at room temperature. After mixing it well, it was centrifuged at 7,000 x g for 10 min to separate into two distinct phases, the upper hexane and the lower aqueous phase containing glycerol. Similar procedure was repeated three times with hexane and consequently, three grades of solvent washed glycerol were obtained. Amount of glycerol was analyzed by HPLC. Inhibition of Crude Glycerol on the Growth of C. freundii The purpose of this study was to determine the in vitro susceptibility of C. freundii to glycerol obtained from different sources. Inhibition due to impurities in crude glycerol was studied by using test tubes containing 9ml of culture medium with different grades of glycerol. Medium was inoculated with 1 ml of inoculum and incubated at 300C. The optical density at 650nm (OD650nm) was measured after 6h in each tube. The percent inhibition was determined from the following equation: Where, c means control tube devoid of glycerol and [ODc(t=6h) - ODc(t=0)] shows the increase of OD650 in absence of glycerol. Ei means experiment containing either pure, crude and pretreated glycerol and [ODEi(t=6h) – ODEi(t=0)] shows the increase of OD650 in presence of glycerol. Scale up at 30 L Bioreactor Scale-up was carried out in a 30 L bioreactor (Scigenics, India) with 22L working volume of the optimized medium (pH 7.0). Prior to inoculation, strict anaerobic conditions were established with sparging with C02. The production medium was sterilized at 1210Cfor 15 min and was inoculated with 2% (v/v) of the inoculum with an absorbance at 0.7 at 660nm. Fermentation was carried out at 300 ± 10Cwith carbon dioxide flow rate of 0.5 volume of air per unit volume of the medium (vvm) at an agitation rate of 200rpm. Foaming was controlled by adding silicon antifoam agent (0.1ml of 50%, v/v, prepared in distilled water). Samples were withdrawn periodically at an interval of 6 h till 60h and analyzed for 1,3-propanediol, lactic acid and acetic acid concentration. Analytical Procedures Estimation of Biomass After desired incubation period, the culture was centrifuged at 10,000 x g for 15min (Sigma centrifuge). Cell pellet was used for growth estimation. Here, pellet was washed with 2 ml of distilled water and again centrifuged at 10,000 x g for 10 min. the process was repeated three times to remove all media components from the cell surface. Now, the pellet was re-suspended in 1 ml of double distilled water and the absorbance was read at 660nm against control using spectrophotometer (UV-Visible 1700, Shimadzu Corp., Kyoto, Japan). Estimation of Fermentation Byproducts After the desired incubation period, the culture was centrifuged at 10,000 x g for 15min (Sigma centrifuge). Supernatant thus obtained was filtered through nylon syringe filters (pore size 0.45 μm, Mdi filters, India). Samples thus obtained were analyzed for the estimation of glycerol, 1,3-propanediol, lactate and acetate concentration by a high-performance liquid chromatography system (Shimadzu 10AVP, Shimadzu Corp.. Kyoto, Japan) equipped with Aminex HPX-87H column (300mm × 7.8mm) (Bio-Rad, Palo AHO, CA, USA) with RID-10A refractive index detector. The working conditions were: 5mM H2SO4 as a mobile phase with a flow rate of 0.6 ml/min and 600Cas the working temperature. Estimation of Free Fatty Acids and Fatty Acid Methyl Esters The estimation of methyl esters were analyzed by a Gas chromatography system (GC-2014, Shimadzu Corp., Kyoto, Japan) equipped with a capillary column (Stabilwax-DA, 30m x 0.32 mm, 0.25 μm film thickness) and flame ionizing detector (FID) was used. Helium gas was used as the carrier gas at a flow rate of 2.87 ml/min. Injection was done in spilt mode (1/5) and the injector and detector and oven temperature were at 250, 260 and 1600C, respectively. Samples (1 μm) were injected, then the oven was heated at 40C/min to 2500C(holding for 10 min). The samples were prepared by filtration using nylon syringe filters (pore size 0.20 μm, Mdi filters, India). Top Results Glycerol From Biodiesel Via Transesterification of Various Oils Biodiesel was synthesized by transesterification reaction using Jatropha, Soyabean, Sunflower, Kardi, Linseed and Rice bran oils, at oil:methanol:: 1:4 molar ratio. The glycerol concentrations in the bottom phase obtained after biodiesel preparation are given in the Table-1. Highest concentration of glycerol was achieved from sunflower and linseed oils. Formation of soap layer was observed in jatropha oil transesterification. Fermentation of Glycerol Obtained From Different Sources The glycerol samples, separated from biodiesel layer by the separatory funnel, were dissolved in distilled water, such that the glycerol concentration of each stock solution becomes 200g/L. After an hour, the white precipitates formed at the top of the stock solution, potentially the potassium and sodium salts of free fatty acids. Similar was observed by Celik et al. [9] while working with glycerol obtained from transesterification of canola, soyabean and sunflower oils. As obtaining a clear solution is necessary for proper spectrophotometric measurement of cell concentration, precipitate was filtered through Whatman filter paper no. 1. Clear solutions were obtained mixed with the production medium, so that the initial glycerol concentration was 50 g/L in all of the media. The inhibitory effect of various types of raw glycerol and of commercial glycerol (denoted by oil abbreviation suffix by C) at a concentration of 50g/L on the growth of C. freundii is presented in Fig-1. Even with the pure glycerol, 23% of growth inhibition was observed. The percentage of growth inhibition increased significantly by using crude glycerol. The growth inhibition was very profound (76%) when KR-C glycerol was used. Inhibition percentage by SB-C, SF-C, LN-C and RB-C were very similar which is approximately in the range of 50–55%. The growth of C. freundii showed a prolonged lag phase, not accompanied by significant substrate uptake when using crude glycerol. Crude glycerol contains free fatty acids, methanol and soap impurities which may interfere with cell division, consequently reducing it. Apart from that raw glycerol often contains a sodium and heavy metals ion which reduces the cell viability [10]. To examine if crude glycerol can be used directly in 1,3-propanediol production, a batch fermentation was conducted and for comparison, commercial glycerol was used as a control experiment. The results of batch fermentations are shown in Table-2. The molar yield of 1,3-propanediol obtained was 0.66 per gm of pure glycerol which is higher than any of the crude glycerols used. Due to the growth inhibition, the glycerol consumption was incomplete and approximately 14.5 g/L, 14g/L, 13g/L and llg/L of 1,3-propanediol was produced while using RB-C, LN-C, SB-C and SF-C glycerol, respectively. Lowest yield was obtained in JT-C and KR-C. In order to overcome this, treatment of glycerol was performed by washing it with hexane, a non-polar solvent. Effect of Hexane Washing Washing of crude glycerol with solvent resulted in removal of impurities at each step. Amount of glycerol in crude form was considered 100% and similarly the percentage glycerol left in the aqueous phase was calculated. Rehman et al. [11], developed a pre-treatment process to get rid of most of the inhibitory substances present in the raw glycerol obtained from sunflower. As the fatty acid content vary in different oils, so it is important to investigate different oils commonly used for biodiesel production. Glycerol obtained from soyabean and sunflower contained the least impurities which were removed just by first washing and resulted in 94 and 84% of glycerol, respectively. Soyabean and sunflower oils comprised mainly of linoleic acid (52–62%). It can be assumed that impurities mainly comprised of linoleic acid can easily be removed by washing crude glycerol with hexane. Following them linseed and rice bran proved to be a good source that resulted in the 65.4 and 63.7% of glycerol after second washing where impurities mainly consisted of linolenic acid and oleic acid, respectively. The most impure sources were Jatropha and kardi which after three subsequent washing finally resulted in 52 and 40% of glycerol, respectively. However, glycerol was also lost to a certain amount in each step of washing Inhibition of Hexane Washed Crude Glycerol on the Growth of C. freundii The inhibitory effect of different grades of hexane washed crude glycerol (denoted by oil abbreviation suffix by H and no. of washing) was evaluated. The results are presented in Fig-2. The commonly found free fatty acids in the crude glycerol obtained from various oils were palmitic acid, stearic acid, oleic acid, linoleic acid and linolenic acid. Saturated free fatty acids do not usually interfere with bacterial growth, however, unsaturated free fatty acids (particularly oleic and linoleic acids) have profound influence on the viability of bacterial cells which is due to the instantaneous depolarization of cell membrane [12]. In the present investigation, it was observed that RB-H showed remarkable reduction of inhibition percentage even afterlst washing. RB mainly comprised of palmitic, oleic and linoleic acids which easily got washed with the hexane. However, JT with similar composition showed profound inhibition even after 3rd washing which is potentially due to the significant soap formation. RB-H was followed by SF-H and SB-H which showed gradual reduction of growth inhibition after 2nd washing. Linoleic acid forms the main constituent of both the oils. LN oil comprised mainly of linolenic acid which was found to be inhibitory to the growth of C. freundii. Even after 3rd washing LN-H glycerol showed 35% of inhibition. In spite of three subsequent washing, KR-H glycerol showed no significant change in growth inhibition. Utilization of Different Grades of Hexane Washed Glycerol By C. freundii Here, different types of hexane washed crude glycerol obtained were evaluated and compared for 1,3-propanediol, lactic and acetic acid production. The results are presented in the Table-3. Glycerol stock was prepared similar to the crude glycerol. A direct relationship between cell growth and end fermentation products was observed. RB-H proved to be a promising substrate showed 1,3-propanediol yield equal to 0.51 g/g while the acetic and lactic acid presented yields of 0.161 and 0.14 g/g, respectively after first washing. After second and third washing 1,3-propanediol yield reached to the 0.66 g/g. After RB-H, next glycerol sources which proved to be a good source were SB-H-2 and SF-H-3. LN-H and KR-H in spite of the three subsequent washing showed no significant increase in the production. JT-H also needed at least three washing with hexane to give results comparable to RB-H-1. No significant differences were observed between pure and SB-H-2, SB-H-3, SF-H-3, RB-H-2 and RB-H-3 in terms of yield (g/g) of 1,3-propanediol, hence suggesting that the suitability of the hexane washed crude glycerol for bioconversion to 1,3-propanediol by C. freundii. Scale up at 30 L Bioreactor In this study, fermentation of glycerol was scaled up to 30L bioreactor (Fig-3). The goal of this study was to test a reliable and scalable fermentation process using 50g/L of glycerol. The effect of 50 g/L of pure glycerol was evaluated for cell growth and production of 1,3-propanediol, acetic acid and lactic acid. The initial pH value of the media was adjusted to 7.0. Samples for product analysis were taken after every 4 h of incubation. Significant cell growth and increased 1,3-propanediol concentration were observed. The 1,3-propanediol concentration obtained from glycerol was 28.0g/L in 48 h. Organic acids, such as acetic acid and lactic acid were also produced at the concentration of 8.5 and 6.8 g/L, respectively. Top Discussion It has been reported that crude glycerol obtained through the transesterification process using oil do not support growth of several strains of C. butyricum [13]. It may be due to the fact that industrial glycerol from various fat feed-stocks may differ considerably from commercial glycerol due to the impurities like methanol, free fatty acids, fatty acid esters, soaps and salts. Glycerol obtained in particular through the transesterification process retards the microbial growth rate and sometimes even become toxic to the cell. To achieve maximum yield of biodiesel using various oils, the transesterification reaction was performed at optimum conditions. Best results were achieved with the sunflower, soyabean, linseed and rice bran oils and the least results were achieved from jatropha and kardi oils. When the effect of various types of raw glycerol at a concentration of 50g/L on the growth of C. freundii was evaluated, significant reduction of growth inhibition was observed which further reduced the production of 1,3-propanediol. This decrease was due to the impurities in crude glycerol which mainly consisted of free fatty acids. Remarkable decreases of viability and morphological changes such as loss of cell shape and disruption of cell membrane were observed in bacteria exposed to unsaturated free fatty acids [14,15]. The growth of bacteria used to assimilate free fatty acids from vegetable oils was also restricted by unsaturated fatty acids, particularly linoleic acid [16]. 1,3-propanediol production is growth dependent so similar production profile was observed in glycerol fermentation. In order to overcome this, washing of crude glycerol was performed. This method resolves the problem of expensive purification processes required for its utilization prior to fermentation. Rehman et al. [11] first time reported a pretreatment process of crude glycerol obtained from sunflower oil biodiesel. In our study, six different oils were used to get a comparative analysis because different oils differ in their free fatty acids contents. Subsequently, growth inhibition percentage was evaluated followed by its fermentation to produce 1,3-propanediol. Significant removal of free fatty acids particularly, palmitic, oleic and linoleic acids occurred by hexane washing. Linolenic and ricinoleic acids were found to be inhibitory to the cell growth. However, after removal of significant amount of free fatty acids, growth as well as production increased and reached up to yield (YPD/S) of 0.66g/g. 1,3-Propanediol production was also successfully scaled up to 30L bioreactor with a yield of 28g/L of 1,3-propanediol. |
Top Conclusion Our strain of C. freundii, efficiently used the crude glycerol, especially after pretreatment. However, we observed a slight decrease in the percentage conversion into 1,3-propanediol while using crude glycerol without any pretreatment. The results obtained showed clearly a resistance to crude glycerol as compared to the solvent-washed glycerol by C. freundii. |
Top Figures Figure 1.: Growth Inhibition of C. freundii by different types of glycerol obtained from various sources
| 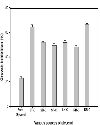 | |
| Figure 2.: Growth Inhibition of C. freundii by different types of hexane washed glycerol obtained from various sources
| 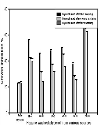 | |
| Figure 3:: Scale up of glycerol fermentation at 30 L bioreactor
| 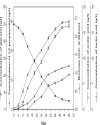 | |
|
Tables Table 1:: Concentration of glycerol in the bottom phases obtained from transesterification reaction of various oils
| Oil used for transesterification | Volume of bottom phase (ml/L) | Concentration of Glycerol in the bottom phase(g/L) | Sample code | Jatropha | 54ml | 500 | JT-C | Soybean | 65ml | 504 | SB-C | Sunflower | 70ml | 892 | SF-C | Linseed | 62ml | 882 | LN-C | Rice bran | 72ml | 640 | RB-C | Kardi | 40ml | 478 | KR-C |
| | Table 2:: Molar yield and inhibition percentage of 1,3-propanediol production from crude glycerol against pure glycerol.
| | Source of Glycerol | 1,3-propanediol | Pure Glycerol | JT-C | SB-C | SF-C | LN-C | RB-C | KR-C | YPD/S (g/g) | 0.66 | 0.16 | 0.26 | 0.22 | 0.28 | 0.29 | 0.14 | Inhibition percentage against pure glycerol(%) | - | 75.7 | 59.6 | 66.6 | 64.2 | 56.0 | 78.8 |
| | Table 3:: Molar yields and inhibition percentage of 1,3-propanediol, acetic acid and lactic acid from hexane washed glycerol against pure glycerol
| Source of Glycerol | 1,3-propanediol | Acetic acid | Lactic acid | | YPD/S (g/g) | Inhibition | YAA/S (g/g) | Inhibition (%) | YLA/S (g/g) | Inhibition (%) | Pure Glycerol | 0.66 | - | 0.17 | - | 0.10 | - | JT-C | 0.16 | 75.7 | 0.081 | 52.3 | 0.06 | 40 | JT-H-1 | 0.40 | 39.2 | 0.13 | 23.5 | 0.11 | 0 | JT-H-2 | 0.52 | 21.2 | 0.15 | 11.7 | 0.10 | 0 | JT-H-3 | 0.63 | 4.5 | 0.17 | 0 | 0.10 | 0 | SB-C | 0.26 | 59.6 | 0.092 | 45.8 | 0.059 | 41 | SB-H-1 | 0.48 | 27.3 | 0.14 | 17.6 | 0.08 | 20 | SB-H-2 | 0.59 | 10.6 | 0.16 | 0.05 | 0.10 | 0 | SB-H-3 | 0.64 | 3.0 | 0.18 | 0 | 0.11 | 0 | SF-C | 0.22 | 66.6 | 0.087 | 48.8 | 0.07 | 30 | SF-H-1 | 0.34 | 48.5 | 0.11 | 35.3 | 0.12 | 0 | SF-H-2 | 0.48 | 27.3 | 0.18 | 0 | 0.14 | 0 | SF-H-3 | 0.54 | 18.2 | 0.20 | 0 | 0.16 | 0 | LN-C | 0.28 | 64.2 | 0.11 | 35.3 | 0.066 | 34 | LN-H-1 | 0.32 | 51.5 | 0.88 | 41.8 | 0.07 | 30 | LN-H-2 | 0.38 | 42.4 | 0.10 | 41.1 | 0.07 | 30 | LN-H-3 | 0.40 | 39.4 | 0.11 | 35.3 | 0.08 | 20 | RB-C | 0.29 | 56.0 | 0.096 | 43.5 | 0.068 | 32 | RB-H-1 | 0.51 | 23.0 | 0.161 | 5.5 | 0.14 | 0 | RB-H-2 | 0.64 | 3.0 | 0.177 | 0 | 0.10 | 0 | RB-H-3 | 0.66 | 0 | 0.173 | 0 | 0.10 | 0 | KR-C | 0.14 | 78.8 | 0.084 | 50.5 | 0.08 | 20 | KR-H-1 | 0.28 | 57.5 | 0.092 | 45.8 | 0.14 | 0 | KR-H-2 | 0.42 | 36.4 | 0.12 | 29.4 | 0.16 | 0 | KR-H-3 | 0.48 | 27.3 | 0.14 | 17.6 | 0.18 | 0 |
| |
|